Viewpoints Concerning the Dependency of Viticulture on the Environment
The determining and limiting properties of the environment which have an effect on the plants vary in nature, and are the subject of multifaceted studies in various disciplines, including climatology, geology, soil sciences, botany, biology, hydrology, etc., determining the behaviour and the overall pattern of the crop (metabo‑ lism, growth, etc.).
As previously mentioned, guidelines have been established which determine the relationship and interaction between the environment and the vine, where “natural media” (collectively, the environment) are some of the most important variables affecting the quality of the grape. This is the core of the idea of terroir.
The natural media, moreover, provide the preconditions for originality. There are some regions of the world which produce better wines than others, where in certain years outstanding wines have been produced by some, and less good wines by others. The natural preconditions which brought about this special quality in a particular area are difficult to duplicate in that same area, and practically impossible to duplicate in any other area, even if the latter has similar cultivation conditions.
It is simpler to observe the influence of one single variable; in such a case one can see that a biological process takes place within parameters, with maximum and minimum values and the optimum range in between. Biological processes, however, do not depend upon a single determinant; rather there are interactions which are responsible for these variables having very different outcomes depending on the circumstances. Thus the agricultural “principle of minimum intervention” has become a much broader “principle of limiting factors”.
In the following paragraphs, the most important variables involved in viticulture, and the production and quality of wine will be examined. Individually these would be climate, and meteorological factors (such as temperature, rainfall, etc.) and the environmental factors influencing the soil (geology, geography, geomorphology, and topography). Soil and crop issues will be dealt with in the next chapter.
Climate
Achieving the desired perfection and harmony in the grape, and therefore producing a quality product, is, as a rule, only possible with an optimum climate. This is is not easy, however, because, apart from anything else, the climate‑production‑quality relationship is different for all grapevine varieties.
To define and quantify the climate as the result of meteorological and geographical elements of a location demands prerequisites that are difficult to predict; moreover, it is not possible for certain climatic (meteorological) conditions to occur in the same form in time and space. In this respect, the relationship between properties of the environment (medium) and some characteristic values sought in the quality of the product, which has produced a multiplicity of (bio) climatic indicators (see below), led to the classification of properties that are characteristic of viticultural regions. This generally led to an assessment of the conditions which need to be put in place to ensure proper maturation of the grape.
The geographical locations around the world where viticulture is practised provide an obvious example of climatic limitation. It is clear that temperature distribution occurs in parallel bands around the globe (thermal zones). The limits for viticulture lie at latitudes 50° N and 40° S. The varying suitability of these regions reflects the confining nature of climate. For example, “Wagner’s line” divides the continent of Europe into two, with one zone in the North being influenced by the Atlantic climate, where wines with low alcohol content but high acidity and pronounced fruitiness are produced, and the other zone in the South with climatic influences from the Mediterranean producing wines with high alcohol content, low acidity, and aromas which may be enhanced through the ageing process.
In the Northern climatic zone, the limiting factors have a lot to do with available energy levels, i.e. temperature and sunlight, whilst in the Southern regions all thermo‑ energy level requirements are met and vegetative activity is sensitive to prolonged dry periods, something which is more pronounced in regions with warm climates where rainfall deficit occurs in the warm months.
There is extensive literature on the subject of climate and viticulture (Coobe, B.G. 1987; Riou, et al., 1992; Becker, W et al., 1994; Wilson, 1998, etc.). The brief assessments made here will be confined to illustrating the impact of climate on viticulture.
Energy and Temperature
The grapevine needs a lot of sunlight and its photosynthesis activity increases with the wavelength of the visible spectrum. Together with thermally satisfactory conditions (25‑30° C) the optimum lies between 30,000 and 60,000 lux (1000 W/m2 = 96,000 lux); when the temperature rises above 30° C and visible light exceeds 100,000 lux, the stomata of plants close.
The intensity of photosynthesis, and therefore the growth of shoots, is closely related to temperature, achieving maximum benefit between 25° C and 30° C. Likewise, the speed of growth depends on the photophase, and increases when the length of the day increases to thirteen hours; a great intensity of light (up to a certain limit) promotes the growth and maturity of the grapes.
Light and temperature (Fig. 131) play a decisive role in pollination in that they facilitate the inflorescence differentiation process; temperatures of 20‑25° C and dry conditions promote flowering. Cool and wet weather conditions lead to poor fertilisation (and millerandage). Temperature and light intensity promote the growth and maturity of the berries, by increasing photosynthesis activity, and therefore stimulating sugar formation, colour synthesis, the aroma components, and lastly, acid reduction. Usually, the asynchronised development of berries and bunches is caused by their different exposure to light, which then manifests itself in uneven development of polyphenols and dry matter in greater shading. At 10° C vegetative growth begins; bud burst begins in the spring at this ambient temperature, and ends in October when the temperature falls below 10° C again. Depending on the grapevine variety and the region, shooting begins at temperatures ranging from 9° C to 13‑14° C. This means that root system activity begins earlier and ends later because a soil temperature of 10° C is achieved earlier in Spring and lasts longer in Autumn.
Extremely high temperatures also have significant effects on the crop. Temperatures over 42° C pave the way for drought damage, with leaves and grapes drying out. At temperatures over 55° C the dried out organism dies (Fig. 136).
At the opposite extreme, plants suffer damage at low temperatures, and their vegetation can be destroyed too. If during flowering there is frost at ‑0.5° C for over half an hour, fruit set is compromised; at ‑1.1° C for half an hour the fruit freezes, whilst one hour at ‑2.5° C will freeze the herbaceous organs. In October with temperatures at between ‑8° C and 12° C, severe organ damage can occur, and if winter temperatures reach between ‑16° C and ‑20° C even those organs in hibernation may be destroyed (Fig. 136).
Fig. 131 shows the distribution of daylight hours, and Fig. 132 the average annual temperatures on the Iberian Peninsula. A clear correlation can be drawn between these maps and those showing the designated quality wine regions (Fig. 124). All D.O. (designated origin) areas are in the 10° C+ isotherm, and receive over 2,000 hours of sunlight, with the highest concentration of vineyards in those areas receiving more than 2,600 hours of sunlight.
Rainfall
Rainfall is one natural phenomenon that is crucial to the development of the vine, because it plays a significant role in determining the quantity and quality of grapes. In combination with temperature and soil moisture, it determines how much water is available to the plant. In effect, this is an interaction between climatic and edaphic factors.
Water requirements depend on the quantitative and qualitative demands of production. Traditionally, additional irrigation has been thought of as advantageous if the total water supply during the vegetative phase is less than 360 mm, but considered unnecessary if it exceeds 500 mm. It is generally accepted that the vineyard needs 300‑ 500 mm rainfall from bud burst to veraison, and between 250 and 700 litres of water for the production of one kilogram of dry matter, which makes a total of between 700 and 1,000 litres per vine.
Lack of water reduces growth and yield. Summer heat shortens the ripening period, reduces acid, colour intensity and sugar content, and promotes drying out. By contrast, excessive rainfall can cause pest damage and increases the likelihood of the occurrence of fungal diseases such as Peronospora and Botrytis. It can also cause soil erosion, and if drainage is insufficient, kill the roots, or lead to coulure at flowering, or splitting of the berries during veraison.
The average rainfall on the Iberian Peninsula is shown in Fig. 133. If one compares these maps with the distribution of the D.O. wine growing regions (Fig. 124), one can deduce that 700 mm rainfall is a good upper limit for the cultivation of quality wines, even if the relationship is not as well defined for rainfall as it is for temperature. Minimum rainfall is not significant in that too little rainfall is easier to correct through the use of supplementary irrigation.
Other Climatic Components
Relative humidity, wind, and hail stand out as being worthy of mention amongst the many meteorological components relevant to viticulture.
Optimum photosynthesis occurs at a relative humidity of between 60% and 70%. Lower humidity could inhibit growth, particularly if combined with a water deficit, which is why it is interesting to study periods when relative humidity falls below 40%. The observation of high humidity (over 80%) from a phytosanitary perspective is also of interest because of the risk of cryptogamic infections, even if in very particular cases such damage was caused by excessive water.
Wind is an important factor to consider for two reasons: first, because it can work to advantage or disadvantage on other meteorological components (humidity, frost, diseases, etc.); and second, because its direct physical action is responsible for the loss of grapes, leaves and inflorescences. The only effective protection is the construction of wind breaks, which entails the expense of building and maintaining them (Fig. 137). Hail affects plants in other ways. Depending on the stage in the growth cycle, it can destroy buds and shoots; later the leaves and flowers are the most susceptible, and hail can cause heavy losses to a growing canopy, but by stripping secondary buds from the old wood and the remaining shoots some balance may be restored. After drying, in addition to the direct loss and damage to shoots and grapes, partly damaged grapes may still die. The damage to the grape stems can prevent substrate flow and can lead to loss or reduction in quality. The closer to maturity the grapes are, the worse the damage from breakage and rot (Fig. 126).
Evapotranspiration and Humidity Deficit
Potential evapotranspiration (PET) plays an important part in climate analysis because it is the one element which together with rainfall determines humidity deficit or excess.
Although evaporation can be measured directly using various types of evaporimeters, and can be considered as an appropriate mechanism, mathematical methods are most effective in determining the PET. Thornthwaite’s method (1948) determines PET based on average temperatures, adjusted depending on the astronomical time of the day and the number of days in the month. The method is widely used in hydrology and in estimating the hydric balance in respect of the climatology and hydrology of river basins, and also to obtain climatic indicators. This method, however, has serious disadvantages in risk planning and assessment.
Figure 134 shows the differences between the various D.O. areas in terms of PET. Specifically, there are three well‑defined zones: the Northern one extends to the 750 mm rainfall contour line. It follows the Tagus River and is bound in the North by the Iberian Cordillera and the Ebro river basin; the Southern zone extends to the North as far as the 900 mm contour lines and more or less follows the course of the Guadalquivir as far as Valencia; between them is the central zone which receives 750 – 900 mm rainfall annually.
In order to establish the hydric balance, the rainfall deficit is calculated by subtracting PET from actual rainfall. This index is very important from a viticultural perspective, since it shows how various discrepancies, such as the length of the vegetative cycle, for example, affect the quality of the wine. Figure 135 shows its geographic distribution in which we can better profile the zones that concern us.
Organisation and Data Management
Macroclimates, or large, relatively uniform units, can be defined based on meteorological information. Often these units are referred to generically; as an Arctic climate, a moderate (oceanic or continental) climate; or a tropical or Mediterranean climate, for example. But for the purpose of comparison it would seem to be more appropriate to select specific subdivisions that permit a quantification of the desired constituent elements.
The Köppen climate classification system is the most widely used among geographers for this purpose. The basic idea is that vegetation is in itself a good indicator of the climate. Accordingly, different climate types are primarily defined by temperature and average rainfall, and thus grouped by common characteristics into sub‑groups, and then broken down further.
The first climate category is made up of 5 climate groups, each denoted by a capital letter , and assigned characteristics as follows: A – tropical humid; B – dry; C – mild mid‑latitude (moderately humid and temperate); D – severe mid‑latitude (moderately humid and cold); and E – polar. Sub‑groups in each of these categories are assigned a second letter in relation to seasonal rainfall distribution: f – no dry season; s – dry season in summer; w – dry season in winter; m – monsoon; W – desert; S – steppe; T – tundra; F – perennial ice. Lastly, classifications are given regarding the thermal conditions, using the lower case letters a, b, c, d, h, y, and k. On the Iberian Peninsula we find the following climate types: B (BW, BSh, BSk); C (Csa, Csb, Cfa, Cfb); and D (Dsb, Dfb).
A comparison of the Köppen climate map (Fig. 129) with each of the areas of designated origin (Fig. 124), clearly shows that three climate zones are suitable for quality wine production: Bsk ( semi‑arid steppe, cold and dry); Csa (mild mid‑latitude, Mediterranean, with dry, hot summer); and Csb (mild mid‑latitude, Mediterranean, with dry, warm or mild summers).
Bsk denotes a climate with low rainfall, arid or semi‑ arid in nature and moderate temperatures under 18° C, and corresponds to vast areas around Badajoz, the Ebro valley and South of Madrid, as well as the provinces of Toledo and Ciudad Real, the Southern stretch of the Levant and along the coast of the islands of Majorca and Ibiza.
In group C, the mean temperature is colder and ranges between ‑3° C and 18° C. This group has the sub‑categories: Csa (mild climate with dry and hot summers, with average temperatures in the hottest month exceeding 22° C); Csb (mild climate with dry and hot summers with average temperatures below 22° C, and has four months of the year when temperatures do not fall below 10° C); and Cs (mild climate with hotter season in summer), all of which are of great viticultural interest and which cover a large portion of the Iberian Peninsula. The sub‑category Csa takes up the South‑Western region of Portugal, half of Spain, with the exception of the Bsk, and the North of the islands of Majorca, Menorca and Ibiza. The sub‑category Csb features in the North‑West of Portugal, Southern Galicia, Castile and Leon, Central Iberia, and higher altitude regions in East Andalusia.
Within the macroclimatic regions, mesoclimates differ from one another because of the specific nature of certain properties, such as thermal energy retention, and frost risk, and how these influence the composition of the grapevine varieties grown.
Defining mesoclimatic characteristics, i.e. the definition of the local climate by its geographical and geomorphological features (river basin, plateau, mountain, and coast) is reasonably straightforward in the areas under discussion, because of the high number of meteorological stations.
As with the macroclimate, the mesoclimate allows an evaluation of the zoning of specific regions in viticultural terms, although the specific nature of the terroir, or characteristics of the soil, can only be determined by the microclimate.
The microclimate refers to the environment immediately surrounding the vineyard regardless of what is happening beyond it. Viticultural management practices such as pruning, shading, wind protection, irrigation and drainage can modify this microclimate.
There are many indices used to determine the climatic potential of a site, particularly those affecting photosynthetic potential, which depends largely on light conditions, temperature, and availability of water. These include:
• the duration of active period of vegetative growth; the number of days during the active period with temperatures above 10° C (the cut‑off point below which significant vegetative growth does not occur);
• the Active Thermal Integral (ATI), which is the sum of the average daily temperatures above 10° C during the active phase. Depending on the requirements of the grapevine variety, this heat summation value typically ranges between 2,720 and 3,190, and sometimes higher (Puillat and Angel), although other authors perform this calculation differently and arrive at values between 4,000 and 5,000.
• Winkler and Amerine’s Effective Thermal Integral (ETI), which is the sum of effective daily mean temperatures (Te), calculated from the monthly average temperatures multiplied by the days of each month during the growing season from April to October. The effective temperature (Te) is the active temperature (Ta) minus 10° C: (Te = Ta – 10° C). In terms of this integral, there are five zones of varying suitability, with values ranging between >1,371.81 and <2,204.01 (Celsius).
• Huglin’s index of heliothermal aptitude (HI), which is the ratio of the average and maximum daily temperatures during the active growth cycle combined with a longitude coefficient which varies with latitude. The calculation is performed by using the monthly climate data recorded during vegetative growth.
• the Cool Night Index (CI), which is a variable which takes into account the average night‑time temperatures during the month of maturation. The development of fine aromatic qualities in certain varieties is only possible if it is cool at night. The calculation is as follows: CI = average minimum air temperature during September (or March, in the Southern Hemisphere).
• the Dryness Index (DI) gives information on water availability in the soil by recording dryness in any given area for 6 months. W=Wo+P‑Tv‑Es, where “W” is the estimated water reserve of the soil required for a predetermined period in millimetres, “Wo” is the usable water reserve in the soil for the roots (mm), “P” is rainfall (mm), “Tv” is the vine’s transpiration potential, and “Es” is direct evaporation from the soil (mm).
• the Multicriteria Climatic Classification System (Geoviticulture MCC System), (Tonietto & Carbonneau, 2000, 2004 ), which is based on integrating three of the above biometeorological indices (HI, CI, and DI) into the viticultural climate of any region classified and grouped in this way, thereby allowing the system to recognize similar climatic conditions.
The application of this method in Spain and Portugal is illustrated in Figures 138a and 138b.
Location and Environment
In this section, the terms environmental conditions and environmental media are used to describe important components of environmental influence, which obviously do not pertain to the actual description of the climate, the soil and plants, but do form part of the essence of the terroir. What is meant in concrete terms is the geographical situation, the geological elements (lithology and nature of rocks) and the geomorphological elements (landscape, slope, exposure, and orientation), which determine the soil’s particular characteristics, as well as their inter‑ relationships and the changes that they ultimately cause.
The relationship of geology to quality is unequivocal. There is no doubt that the character of the wine is associated with the stratigraphic column (technical term to describe the vertical location of rock in the soil), which influences both the aromatic and flavour characteristics of wines, and their colour intensity. The latter may differ widely depending on the origin of the soils that have formed on different materials. The extensive literature on the relationship between geology and quality and the importance of terroir lithostratigraphy (e.g. Pomerol, 1985; Milson, 1998; Fanet, 2002, etc.) needs no further analysis here.
Geomorphology influences the terroir in important ways: as a designer of the landscape (Asselin & Roussy, 2003) and its formative elements, primarily slope, exposure (light), orientation and altitude. These components influence the vineyard and wine quality in subtle ways, and their interactions with each other are significant.
Since the Roman Empire, topography has been considered as having a distinctive influence on the wine. More precisely, slopes, determined by their exposure, orientation and inclination, are important predictors of quality in cool regions, where temperature ranges match the needs of the grapevine variety. Orientation of the slope in relation to the sun determines frost risk, sun exposure and thermal energy levels, as well as the type of soils and availability of water, and therefore influences the formation of berries and the maturation process (phenology) and ultimately, the quality of the wine.
Generally, altitude – especially in conjunction with warmth – exerts an influence on precocity and wine quality. In this regard, various authors tend to associate a particular zone at high altitude with quality criteria and lower altitudes with the loss of complexity and sustainability, and moreover, assert that vineyards which face North produce wines of greater complexity, although in damp and chilly years, a southerly orientation fares better.
Topography
The viticultural regions being examined here consist of territory with Atlantic and Mediterraean influences.
The Iberian Peninsula is situated between the Northern latitudes of 36°00’08” and 43°47’36” and between the Western latitudes of 3°19’05” and 9°30’02” (excluding the islands).
Its relief is characterised by significant contrasts not only in terms of its historical geological structures, but also in terms of the differences in its climatic dominion; the Atlantic Ocean on the one hand and the Mediterranean Sea on the other. On the land mass in between, the mountain ranges change with marked relief into sedate and gentle shapes at medium altitude, separated by depressions which sometimes develop into deep valleys, which are definitely suited to viticulture.
In describing the relief, it is important to note two of its characteristics: altitude and orientation. The average vineyard altitude on the Iberian Peninsula is 650 metres, exceeded only by Switzerland. France’s highest vineyard altitude, including vineyards in the French Alps is 340 metres. The high altitude is due in large part to the central highlands (Northern and Southern Meseta Central) which slopes towards to the Atlantic at a gentle inclination of 3%. From the central highlands the slope also runs in gentle undulations down to the lowlands of the Ebro and Guadalquivir rivers.
The relief is characterized by several mountain ranges and massifs which border on the Meseta Central: to the North‑West, the Galician Mountains with their extension into Portugal by way of the Serras de Marão and Montemuro; to the North and North‑East, the Cantabrian Mountains and the Pyrenees (1,000‑2,500 m) and the Mountains of Catalonia on the coast; to the East the Iberian System in a NW‑SE direction; and to the South, the Baetic Cordillera (3,481 m) and the Sierra Morena. In other words, the central system formed by the mountains of Toledo extends into Portugal, where it forms the Serra da Estrela.
The difficult orography affords unusual conditions which gives rise to the specificity and variability of geographical locations, leading to specific ramifications for its climates. Comparison of the relief map of the Iberian Peninsula (Fig. 140) with the distribution of quality viticultural areas (Fig. 124) shows that three very different situations exist, which lead to particularly meaningful regional and supra‑regional characteristics. To be more precise, the location, direction and particular orographical features divide the Atlantic influence from that of the Mediterranean, the coastal regions from the continental ones, and high altitude regions from low‑lying ones.
The D.O.Ca Rioja and D.O. Ribeira del Duero regions are good examples of the above. The former reveals that the cultivation of the Tempranillo grapevine variety does not occur above an altitude of 600 m, but that differences exist between the Northern and Southern region in that in the latter D.O. region cultivation occurs above 1,000 m. In terms of its behaviour under extreme continental features, the Tempranillo provides an interesting example when compared with the Verdejo D.O. Rueda because of the different expression of its special features.
Lithology
Other elements of the environment play an essential role in the distribution of vineyards, such as the type of rock, with special consideration given to lithology as it relates to the geological past. In this sense and also within the morphological outlines described above, the three main zones can be distinguished as follows: the Variscan Belt (or Hercynian) highlands, the Alpine Belt, and the lowlands (Fig. 139). The Hercynian cratonic block, also known as the Iberian Massif, is located in the Western portion of the Peninsula and includes the catchment areas of the Sierra Morena, the Serra da Estrela, the Mountains of Toledo and the Spanish Central System. In the main, it consists of older silicate‑rich material, primarily from igneous rock such as granite and metamorphic rocks such as slate, gneiss, mica and quartzite.
If one compares this scenario (Fig. 139) with the map showing quality wine regions (Fig. 124), it can be ascertained that regardless of the presence of the grapevine almost everywhere, the majority of the D.O. regions (Fig. 124) lie on the Central System of the Iberian Peninsula, in the Northern and Southern high plains and the foothills. In addition, vineyards exist in the basins of major rivers, and in each of these valleys a different orientation and solar exposure (sun) prevails, giving rise to significant climatic differences, different rock composition and soil landscapes. Vineyards can also be found in certain coastal areas, thus their habitats range from sea level to altitudes of up to 1,800 m (Tenerife).
In summary it can be said that latitude, longitude and altitude, in conjunction with diverse situations, locations, rock types, and landscapes, combined with an enormous varietal richness have provided the Iberian Peninsula with a multiplicity of terroirs, resulting in the widest variety of wines in the world.
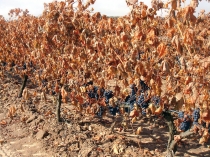
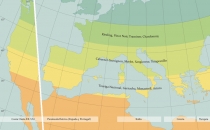